Biological Membranes
Biological cells consist of water-filled compartments enclosed by lipid bilayer membranes. Due to their chemical makeup, these membranes do not readily allow water and water-soluble substances to pass through into or out of the cell. This compartmentalization played a crucial role in the emergence of life on Earth, as it allowed reactants to remain in place for chemical processes.
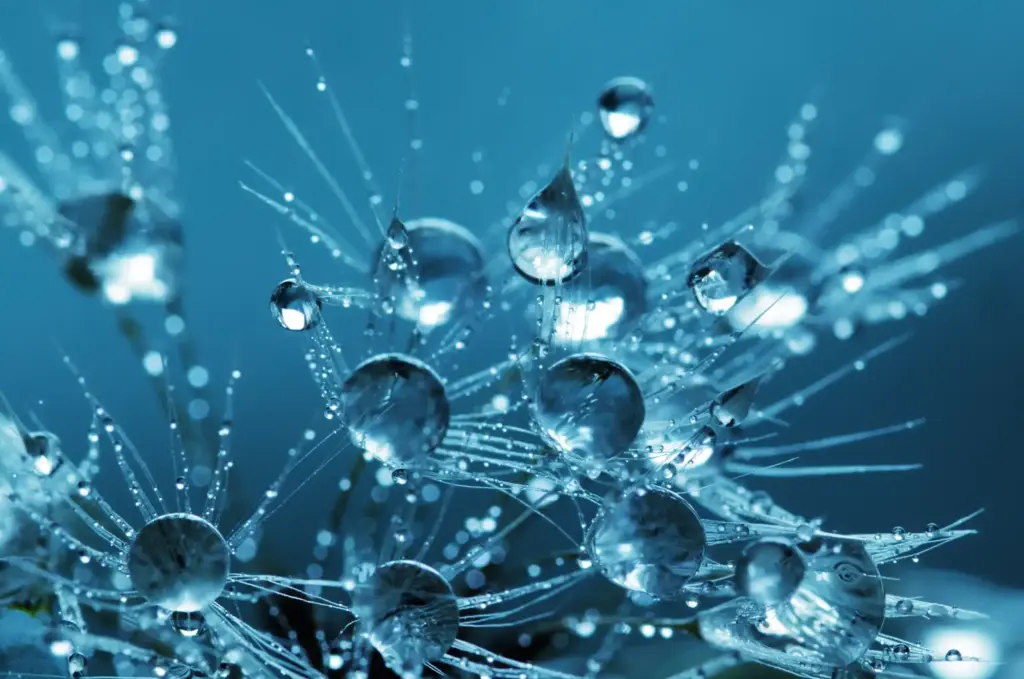
However, chemical reactions quickly come to a halt when reactants are used up. Therefore, in addition to compartmentalization, the earliest living cells also evolved the ability to exchange specific reactants with their surrounding environment to sustain vital (bio)chemical reactions such as energy generation and reproduction (metabolism).
To achieve this, cells developed channels and transport proteins that cross the lipid bilayer, allowing controlled movement of chemicals in and out of cells. Although lipid membranes restrict the movement of water, water can still cross them at a rate sufficient to sustain basic cell functions.
The existence of water channels was therefore a subject of scientific debate until the discovery of Aquaporin-1 in 1991, which led to the award of the 2003 Nobel Prize in Chemistry to Professor Peter Agre.
Aquaporin-1 is highly abundant in kidneys, where it contributes to the filtration process that removes metabolic waste products from the blood and concentrates 180 liters of primary filtrate into just 1-2 liters of daily excreted urine. Aquaporins were then also discovered in a broad range of living organisms, from small bacteria to giant Sequoia trees. In the latter, they facilitate water movement from roots to leaves. It was later discovered that aquaporins also transport other uncharged molecules such as glycerol, which is a valuable nutrient for both bacteria and humans.
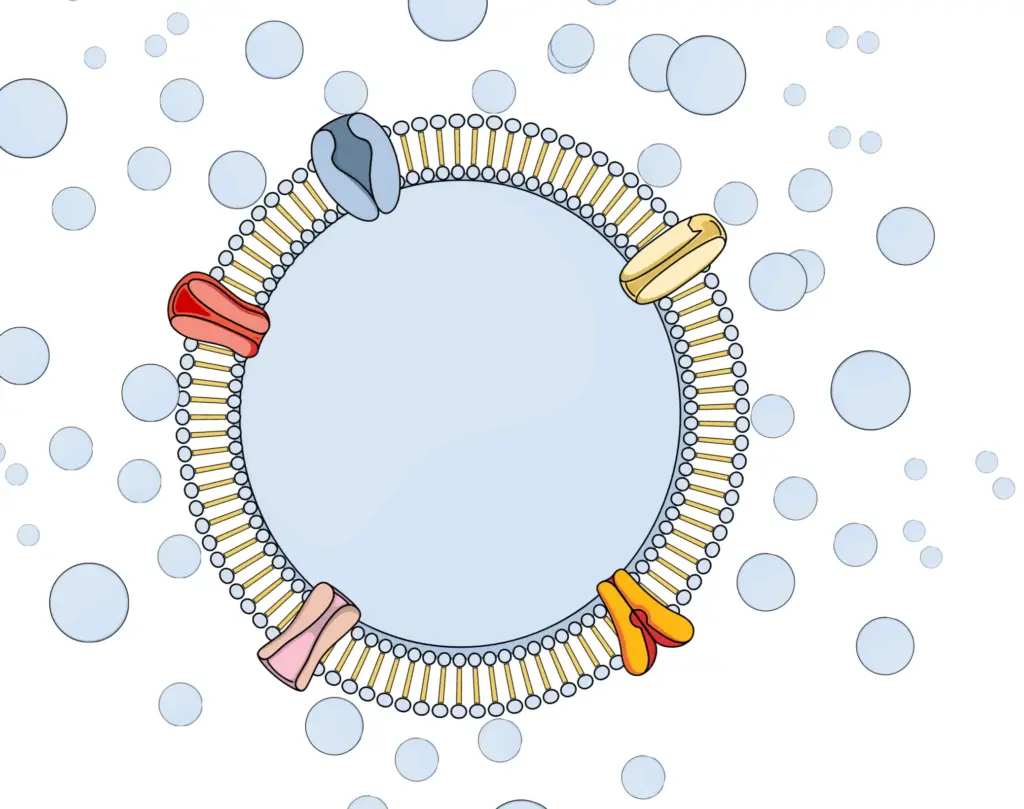
Hydrogen Peroxide: A Key Player in Cell Signaling
Two key characteristics defining life are metabolism and the ability to sense environmental information. For instance, a bacterium can detect the presence of a specific sugar in its environment using a sensor embedded in the outer cell membrane. This external detection often triggers an internal response via the involvement of other molecules.
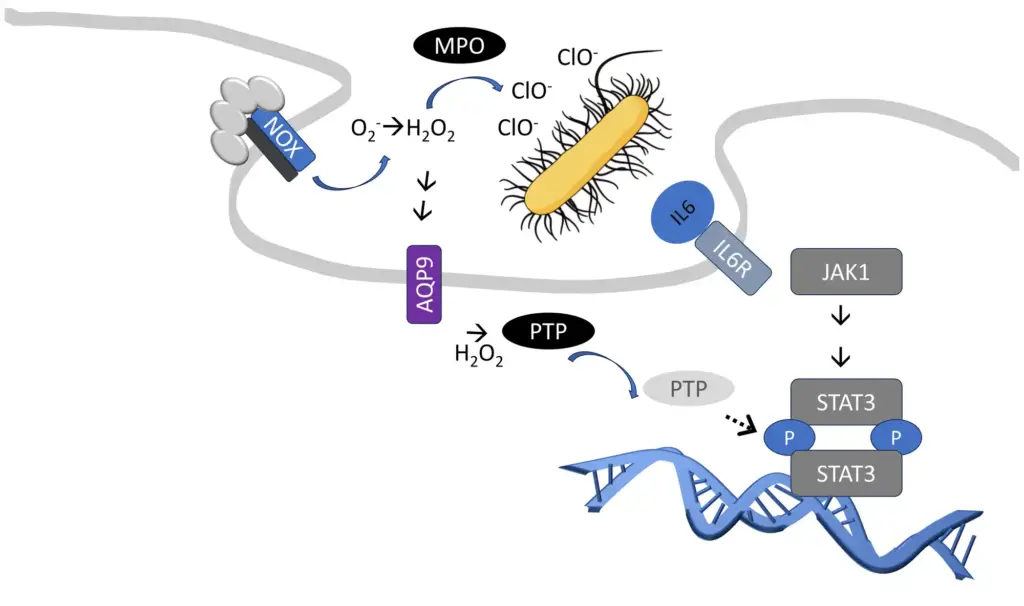
At the other end of the complexity spectrum, the human immune system operates on the same principle. Immune cells interpret chemical information in their environment, such as in the blood, and respond by releasing new information.
This is often in the form of proteins known as cytokines, which can attract other immune cells to an infection site or stimulate their proliferation. Cytokines bind to specific receptors on cell exteriors and trigger corresponding internal responses. Given the nearly infinite number of scenarios and just under 300 known cytokines, much of the response depends on context modification.
One significant context modification mechanism discovered recently involves reactive oxygen species, particularly hydrogen peroxide (2). Hydrogen peroxide can modify cysteine amino acids in proteins, such as protein tyrosine phosphatases (3), which play a crucial role in interpreting extracellular signals intracellularly.
Interestingly, large amounts of hydrogen peroxide are produced during microbial infections at the cell surface. This is an intermediate step in producing hypochlorite or chlorine bleach, a common household disinfectant that immune cells also use for similar purposes. Simultaneously, some hydrogen peroxide enters immune cells through aquaporins (4), reinforcing information about the presence and type of microbial infections (5).
The Crucial Role of Aquaporin-9 in Neutrophils
The human genome encodes thirteen related aquaporins, each expressed in specific cell types and tissues throughout the body. These aquaporins facilitate the transport of partly overlapping but distinct substrates. Neutrophils, the most abundant cell type in the human immune system, predominantly express Aquaporin-9 (AQP9) (6). Among aquaporins, AQP9 is one of the most effective hydrogen peroxide channels (4).
Neutrophils, as part of the innate immune system, play a significant role in the early response to microbial infections. They are usually the first immune cells to arrive at an infection site and thus shape and coordinate much of the initial response.
Neutrophils can produce large amounts of hydrogen peroxide and hypochlorite and release enzymes capable of breaking down protective microbial surface structures.
The exact molecular details linking the uptake of hydrogen peroxide through AQP9, and the release of antimicrobial enzymes are not fully understood. However, ApoGlyx, in collaboration with international academic partners, has demonstrated that AQP9 significantly contributes to neutrophil activation, and production of reactive oxygen species in tissues (7, 8).
The Double-Edged Sword of Neutrophil Activation
Inborn defects in neutrophil antimicrobial functions, such as a lack of hydrogen peroxide production, unsurprisingly lead to increased susceptibility to infections (9). Similarly, in animal models of bacterial infection, neutrophils’ antimicrobial enzymes are crucial for survival (10, 11).
However, in severe infections, neutrophils can be activated beyond the actual infection site, leading to systemic inflammation (12). In these situations, neutrophils can attach to blood vessels throughout the body and infiltrate both infected and healthy tissue. Instead of solely attacking microbes, neutrophil reactive oxygen species and proteases can increase blood vessel permeability, leading to fluid leakage and subsequent edema in surrounding tissue.
Particularly lung edema can increase the distance for gas exchange between lung alveoli and blood vessels, leading to poor blood oxygenation and complications of severe infection, such as Acute Respiratory Distress Syndrome (ARDS). Moreover, fluid loss from blood vessels can lead to impaired tissue perfusion, further oxygen deprivation, and renal and liver failure. Altogether, this may create a fatal situation where the inability of neutrophils to contain an infection may lead to widespread organ damage. This condition is known as sepsis. In sepsis, neutrophil activity becomes harmful, and short-term suppression of neutrophil activity, combined with standard-of-care treatment, including antimicrobials, is a recognized therapeutic concept (13).
Neutrophil activation can also be triggered by non-microbial events and conditions that cause tissue damage, such as trauma, burn injury, smoke inhalation injury, toxins, gout, acute pancreatitis (14), acute liver failure, transfusion-related acute lung injury (15), or temporary oxygen deprivation of organs (ischemia), and reperfusion. These conditions can induce uncontrolled cell death, resulting in distribution of intracellular material normally hidden from the immune system.
Some of this material, like DNA, RNA, and mitochondria, resembles viral and bacterial infections and may therefore trigger similar neutrophil responses, also known as sterile inflammation.
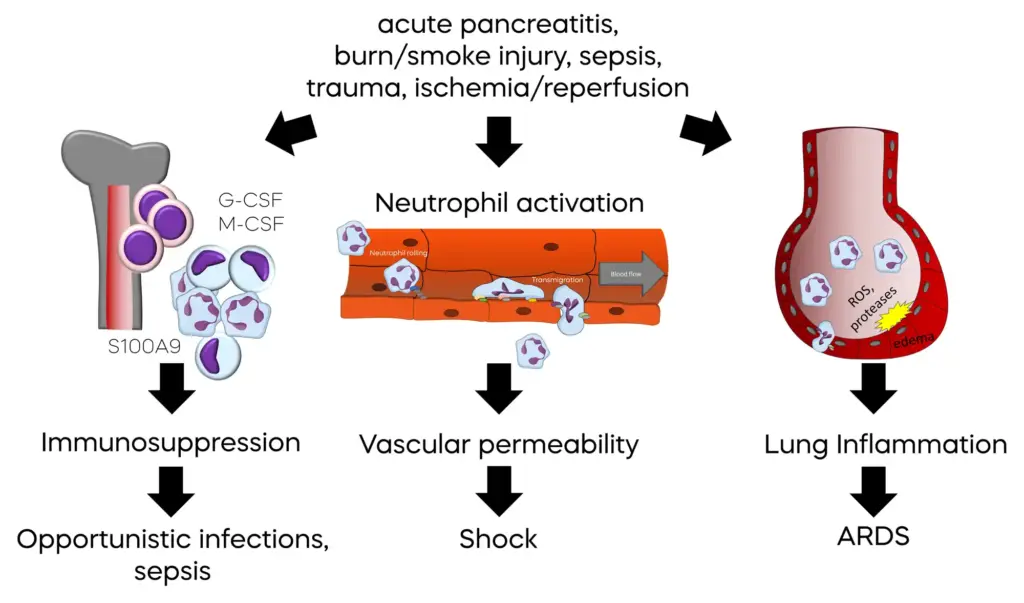
Complicating matters further is that inappropriate mass activation of neutrophils leads to an emergency production of new neutrophils. However, these new neutrophils are released from the bone marrow in an immature state, as myeloid derived suppressor cells (MDSCs). Again, the formation of large amounts of reactive oxygen species during severe inflammation plays a key role in MDSC differentiation (16). These cells lack the necessary functions to fight off microbial infections and can suppress functions of the adaptive immune system, which is responsible for definitive clearance of infections and protection from re-infections with the same pathogen. Consequently, a significant proportion of mortality associated with severe inflammation caused by infections or tissue injury is due to enhanced susceptibility to secondary infections between days and months after the initial, inappropriate, system-wide activation of neutrophils.
The Potential of Aquaporin Blockers as Novel Therapeutics
The therapeutic potential of aquaporin blockers has long been recognized (17). However, the challenge has been in identifying drug-like chemicals that can inhibit aquaporins by blocking the channel pore.
A breakthrough came from an interdisciplinary cell-based and computational approach. This collaborative effort involved researchers at Aarhus University (Denmark), the Max Planck Institute Göttingen (Germany), and Lund University in Sweden, leading to the discovery of a unique series of drug-like small molecule aquaporin inhibitors (18-20).
ApoGlyx was established following further collaboration between researchers based at Lund University and drug development experts at Red Glead Discovery in Lund. The aim was to translate the initial academic results into therapies for human conditions with high unmet medical need. ApoGlyx now owns the resulting intellectual property (21, 22).
In a series of promising in vivo studies conducted at the renowned William Harvey Research Institute in London, UK, a prototype AQP9 inhibitor demonstrated significant protection against sepsis-induced organ damage (7). The study revealed the impact of AQP9 inhibition on the activity of the hypochlorite-generating enzyme myeloid peroxidase in lung tissue. These results are consistent with a previous study that reported enhanced survival of AQP9-/- knockout mice in a different mouse model of sepsis and toxin-induced systemic inflammation (8), thus supporting target specific effects of the inhibitor.
These studies, along with ongoing research, suggest a uniquely valuable, dual benefit of AQP9 inhibitor treatment: (1) a reduction in system-wide neutrophil activation, preventing severe inflammation complications like ARDS and organ failure, and (2) a decrease in the initial release of reactive oxygen species, leading to improved long-term outcomes due to reduced susceptibility to secondary infections.
These effects are expected to be most beneficial in situations of sterile inflammation, e.g. acute pancreatitis, and burn injuries, where early neutrophil antimicrobial activities are not required.
Of further importance, the deletion of the AQP9 gene, which effectively removes AQP9 function from mice, has minimal effects on the animals’ health. Apart from its functions in neutrophils, AQP9 is expressed in very few organs, with the liver being the second main site of expression (23). In the liver, AQP9 serves as a channel for glycerol and urea (20, 24). Glycerol acts as a precursor for glucose and triglyceride synthesis, while urea is a byproduct of amino acid catabolism.
Interestingly, deletion of the AQP9 gene resulted in improved blood glucose concentration in a mouse model of type-2 diabetes, but it did not affect the blood glucose levels in otherwise healthy mice (25). Moreover, effects on the liver transcriptome and metabolome were hardly detectable (26). In agreement with many other studies, these findings support a very mild impact of AQP9 loss-of-function on mouse health.
Even when the urea cycle was stressed in AQP9-/- knockout mice, either through elevated plasma ammonia levels, a high protein diet, or starvation, no concerning deficits were observed (24). Collectively, these studies provide strong evidence that inhibiting AQP9 will not lead to target-related side effects, especially during short-term use for dampening early damage caused by severe inflammation.
Currently, ApoGlyx has meticulously characterized physical chemistry, pharmacokinetic, toxicologic, and pharmacologic properties of lead AQP9 inhibitors. This rigorous process has resulted in the selection of a lead candidate for entering the IND/CTA enabling pre-clinical development phase.
References: Further Reading
- Morris, R., Kershaw, N. J., andBabon, J. J. (2018) The molecular details of cytokine signaling via the JAK/STAT pathway Protein Sci 27, 1984-2009 10.1002/pro.3519
- Rhee, S. G. (2006) Cell signaling. H2O2, a necessary evil for cell signaling Science (New York, NY) 312, 1882-1883 10.1126/science.1130481
- Bae, Y. S., Kang, S. W., Seo, M. S., Baines, I. C., Tekle, E., Chock, P. B. et al. (1997) Epidermal growth factor (EGF)-induced generation of hydrogen peroxide. Role in EGF receptor-mediated tyrosine phosphorylation J Biol Chem 272, 217-221, https://www.ncbi.nlm.nih.gov/pubmed/8995250
- Tamma, G., Valenti, G., Grossini, E., Donnini, S., Marino, A., Marinelli, R. A. et al. (2018) Aquaporin Membrane Channels in Oxidative Stress, Cell Signaling, and Aging: Recent Advances and Research Trends Oxid Med Cell Longev 2018, 1501847 10.1155/2018/1501847
- Warnatsch, A., Tsourouktsoglou, T. D., Branzk, N., Wang, Q., Reincke, S., Herbst, S. et al. (2017) Reactive Oxygen Species Localization Programs Inflammation to Clear Microbes of Different Size Immunity 46, 421-432 10.1016/j.immuni.2017.02.013
- Lindskog, C. (2015) The potential clinical impact of the tissue-based map of the human proteome Expert review of proteomics 12, 213-215 10.1586/14789450.2015.1040771
- Mohammad, S., O’Riordan, C. E., Verra, C., Aimaretti, E., Alves, G. F., Dreisch, K. et al. (2022) RG100204, A Novel Aquaporin-9 Inhibitor, Reduces Septic Cardiomyopathy and Multiple Organ Failure in Murine Sepsis Front Immunol 13, 900906 10.3389/fimmu.2022.900906
- Tesse, A., Gena, P., Rutzler, M., andCalamita, G. (2021) Ablation of Aquaporin-9 Ameliorates the Systemic Inflammatory Response of LPS-Induced Endotoxic Shock in Mouse Cells 10, 10.3390/cells10020435
- Leliefeld, P. H., Wessels, C. M., Leenen, L. P., Koenderman, L., andPillay, J. (2016) The role of neutrophils in immune dysfunction during severe inflammation Critical care 20, 73 10.1186/s13054-016-1250-4
- Belaaouaj, A., McCarthy, R., Baumann, M., Gao, Z., Ley, T. J., Abraham, S. N. et al. (1998) Mice lacking neutrophil elastase reveal impaired host defense against gram negative bacterial sepsis Nat Med 4, 615-618 10.1038/nm0598-615
- Gaut, J. P., Yeh, G. C., Tran, H. D., Byun, J., Henderson, J. P., Richter, G. M. et al. (2001) Neutrophils employ the myeloperoxidase system to generate antimicrobial brominating and chlorinating oxidants during sepsis Proc Natl Acad Sci U S A 98, 11961-11966 10.1073/pnas.211190298
- Singer, M. (2014) The role of mitochondrial dysfunction in sepsis-induced multi-organ failure Virulence 5, 66-72 10.4161/viru.26907
- Francois, B., Wittebole, X., Ferrer, R., Mira, J. P., Dugernier, T., Gibot, S. et al. (2020) Nangibotide in patients with septic shock: a Phase 2a randomized controlled clinical trial Intensive care medicine 46, 1425-1437 10.1007/s00134-020-06109-z
- Hey-Hadavi, J., Velisetty, P., andMhatre, S. (2023) Trends and recent developments in pharmacotherapy of acute pancreatitis Postgrad Med 135, 334-344 10.1080/00325481.2022.2136390
- Jongerius, I., Porcelijn, L., van Beek, A. E., Semple, J. W., van der Schoot, C. E., Vlaar, A. P. J. et al. (2019) The Role of Complement in Transfusion-Related Acute Lung Injury Transfus Med Rev 33, 236-242 10.1016/j.tmrv.2019.09.002
- Ohl, K., andTenbrock, K. (2018) Reactive Oxygen Species as Regulators of MDSC-Mediated Immune Suppression Front Immunol 9, 2499 10.3389/fimmu.2018.02499
- Verkman, A. S., Anderson, M. O., andPapadopoulos, M. C. (2014) Aquaporins: important but elusive drug targets Nature reviews Drug discovery 13, 259-277 10.1038/nrd4226
- Sonntag, Y., Gena, P., Maggio, A., Singh, T., Artner, I., Oklinski, M. K. et al. (2019) Identification and characterization of potent and selective aquaporin-3 and aquaporin-7 inhibitors J Biol Chem 294, 7377-7387 10.1074/jbc.RA118.006083
- Wacker, S. J., Aponte-Santamaria, C., Kjellbom, P., Nielsen, S., de Groot, B. L., andRutzler, M. (2013) The identification of novel, high affinity AQP9 inhibitors in an intracellular binding site Mol Membr Biol 30, 246-260 10.3109/09687688.2013.773095
- Jelen, S., Wacker, S., Aponte-Santamaria, C., Skott, M., Rojek, A., Johanson, U. et al. (2011) Aquaporin-9 protein is the primary route of hepatocyte glycerol uptake for glycerol gluconeogenesis in mice J Biol Chem 286, 44319-44325 10.1074/jbc.M111.297002
- Evenas, J., Larsson, J., andDreisch, K. inventors; APOGLYX AB (Lund, SE) assignee. Compounds for modulating aquaporins
- Rützler, M. L., SE), Brimert, Thomas (Blentarp, SE), Dreisch, Klaus (Lund, SE), Evenäs, Johan (Lund, SE), Larsson, Joakim (Lomma, SE), inventors; APOGLYX AB (Lund, SE) assignee. Derivatives of 2-(1,2,4-triazol-3-ylsulfanyl)-N-1,3,4-thiadiazol-2-yl acetamide which are useful for the treatment of inter alia diabetes
- Lindskog, C., Asplund, A., Catrina, A., Nielsen, S., andRutzler, M. (2016) A Systematic Characterization of Aquaporin-9 Expression in Human Normal and Pathological Tissues The journal of histochemistry and cytochemistry : official journal of the Histochemistry Society 64, 287-300 10.1369/0022155416641028
- Jelen, S., Gena, P., Lebeck, J., Rojek, A., Praetorius, J., Frokiaer, J. et al. (2012) Aquaporin-9 and urea transporter-A gene deletions affect urea transmembrane passage in murine hepatocytes Am J Physiol Gastrointest Liver Physiol 303, G1279-1287 10.1152/ajpgi.00153.2012
- Rojek, A. M., Skowronski, M. T., Fuchtbauer, E. M., Fuchtbauer, A. C., Fenton, R. A., Agre, P. et al. (2007) Defective glycerol metabolism in aquaporin 9 (AQP9) knockout mice Proc Natl Acad Sci U S A 104, 3609-3614 10.1073/pnas.0610894104
- Spegel, P., Chawade, A., Nielsen, S., Kjellbom, P., andRutzler, M. (2015) Deletion of glycerol channel aquaporin-9 (Aqp9) impairs long-term blood glucose control in C57BL/6 leptin receptor-deficient (db/db) obese mice Physiol Rep 3, 10.14814/phy2.12538